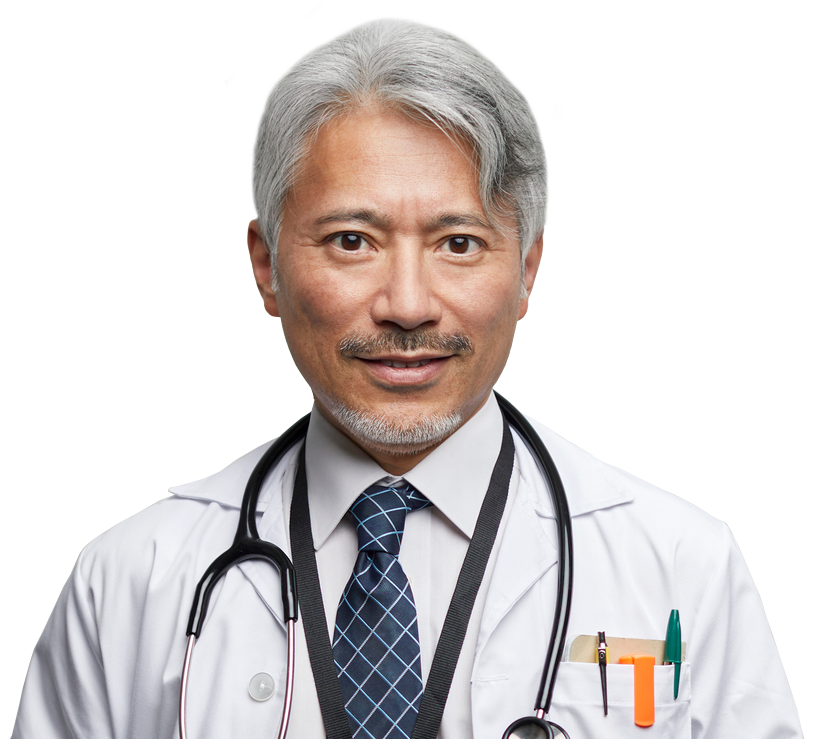
Research Strategies Targeting Dystrophin Production
Though not yet approved by the FDA, several experimental therapies are in various stages of clinical development.
One category of experimental therapies aims to address the cause of DMD, namely, the lack of the dystrophin protein, by restoring production of a functioning form of dystrophin.
Gene Editing
Gene editing is an experimental technique in the very early stages of development.1 The goal of gene editing is to change specific building blocks in the dystrophin gene, like changing letters in an instruction manual, so that cells can produce dystrophin.1 These strategies use a technique called CRISPR/Cas (also called CRISPR/Cas9 or simply CRISPR) gene editing.1
One way that researchers are using CRISPR is to cut out “errors” in the dystrophin gene in heart and muscle cells so that the cells can now read the gene and make dystrophin protein.2 Another potential strategy uses CRISPR to replace the errors in the dystrophin gene with parts from a healthy gene, with the goal of restoring the cell’s ability to produce dystrophin.1
CRISPR gene editing is also being explored in muscle stem cells in Duchenne. Researchers are editing the dystrophin gene in muscle stem cells and investigating whether they can develop into new muscle cells that produce dystrophin.3 The ultimate goal is to regenerate healthy muscle to replace the weakened muscle in Duchenne.